Plant Growth Regulation
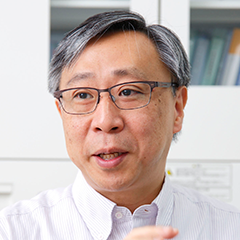
- Professor
- UMEDA Masaaki
- Assistant Professor
- AKI Shiori
- Zhang Ye
- Labs HP
- https://bsw3.naist.jp/umeda/
Outline of Research and Education
Plants continuously produce organs throughout their life. This feature renders them distinct from animals, in which organ formation ceases soon after embryogenesis. We aim to understand the mechanisms of stem cell maintenance, stress response and DNA polyploidization that support sustained plant growth under changing environments. Our study will contribute to the development of technologies to increase plant biomass and food production, and to protect global plant resources.
Major Research Topics
Maintenance and replenishment of plant stem cells
Plants have a long life-span if the developmental program is optimized, and continue to grow throughout their life. This feature is derived from persistent proliferation of pluripotent stem cells scattered throughout the plant body. We are studying the molecular mechanisms of how stem cells are maintained and replenished under DNA stress. We recently found that plant hormones play a crucial role in stem cell death and regeneration; therefore, the focus is on how hormonal signalings are coordinated to preserve stem cells. Genome maintenance in stem cells is also essential for repetitive organ formation, thus we are investigating how hormones control chromatin structure and genome integrity. Our study will contribute to the understanding of mechanisms underlying toti/pluripotency, thereby leading to the development of technologies for modifying plant architecture and enhancing regeneration in tissue culture.
Plant growth regulation in response to abiotic stress
Plant growth is usually inhibited under stressful conditions because plants need to use energy for coping with stress, rather than for organ growth. We have recently identified the signaling cascade that triggers G2 cell cycle arrest in response to multiple stresses, thereby functioning as a module for stress-induced growth arrest. We are studying how this cascade orchestrates the expression of G2/M-specific genes and whether it is conserved among plant species. We are also generating multiple stress-tolerant crops, which can continuously grow under changing environments, by modifying the signaling components.
Mechanisms for induction of DNA polyploidization
In many plant species, cells start DNA polyploidization, called endoreplication, after the cessation of cell division. Endoreplication promotes enlargement of individual cells and organs; thus, it greatly contributes to plant biomass production. While previous studies demonstrated that inhibition of cell cycle progression induces the onset of endoreplication, we recently found that chromatin decondensation can be another cause that triggers endoreplication. We are focusing on the key epigenetic factors controlling endoreplication and investigating the involvement of chromatin dynamics in the transition from cell division to DNA polyploidization. We are also developing technologies to enhance DNA polyploidization in crops and woody plants, aiming to increase food and biomass production.
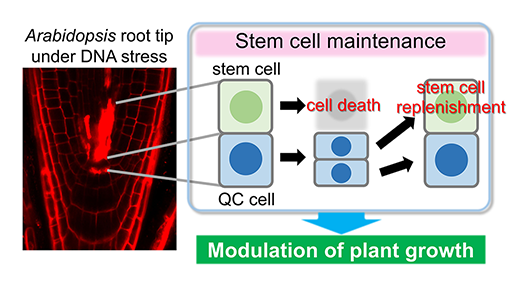
Stem cell death, which occurs in response to DNA damage, is accompanied with the division of a neighboring QC cell, thereby replenishing stem cells.
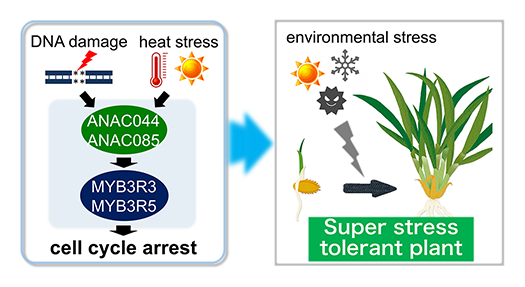
Transcription factors MYB3R3/5 cause G2 arrest in response to DNA damage and heat stress. Suppression of this module will enable us to generate super stress tolerant plants.
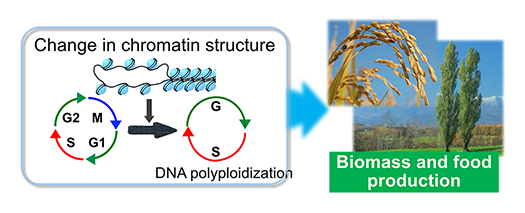
Change in chromatin structure and cell cycle retardation induce DNA polyploidization.
References
- Takahashi N. et al., J. Exp. Bot., 75, 1364-1375, 2024
- Takatsuka H. et al., J. Exp. Bot., 74, 3579-3594, 2023
- Takahashi N. et al., Sci. Adv., 7, eabg0993, 2021
- Shimotohno A. et al., Annu. Rev. Plant Biol., 72, 273-296, 2021
- Umeda M. et al., Plant J., 106, 326-335, 2021
- Watanabe S. et al., Proc. Natl. Acad. Sci. USA, 117, 31500-31509, 2020
- Umeda M. et al., Curr. Opin. Plant Biol., 51, 1-6, 2019
- Takahashi N. et al., eLife, 8, e43944, 2019
- Takatsuka H. et al., Plant Physiol., 178, 1130-1141, 2018
- Ogita N. et al., Plant J., 94, 439-453, 2018
- Chen P. et al., Nature Commun., 8, 635, 2017
- Kobayashi K. et al., EMBO J., 34, 1992-2007, 2015
- Yin K. et al., Plant J., 80, 541-552, 2014
- Takahashi N. et al., Curr. Biol., 23, 1812-1817, 2013
- Yoshiyama K.O. et al., EMBO Rep., 14, 817-822, 2013