The basic function of neurons is to receive signals, integrate them and transmit them to the next neuron. Thus, neurons can function as semiconductors in the brain. Neuronal polarity plays an essential role for this function. Namely, most of neurons form a single axon and multiple dendrites. Dendrites receive signals from outside, while an axon output signals. Then a signal flow arises from dendrites via cell body to the axon. How neurons acquire neuronal polarity? Our laboratory investigates the mechanism for axon/dendrite formation and neuronal polarization, focusing on Shootin1a, Singar and Rab33.
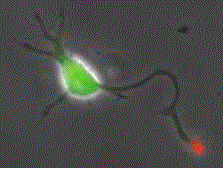
Shootin1a (red) is a key molecule involved in axon formation and axon guidance
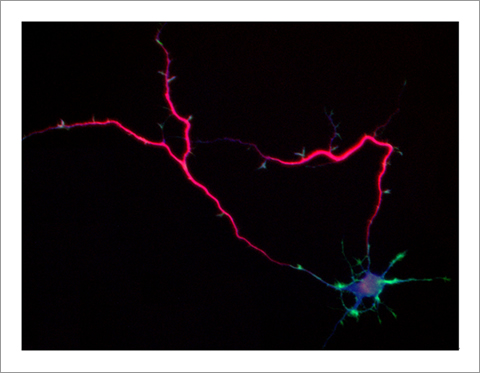
Singar knockdown leads to formation of surplus axons leading to disruption of neuronal polarity
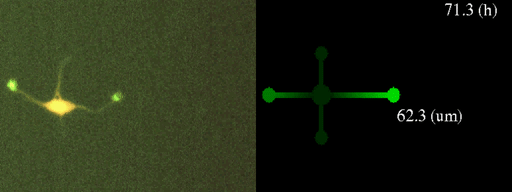
Mathematical modeling of Shootin1-medicated neuronal polarization
Reference
- Huang, L., Urasaki, A. and Inagaki N. (2019)
Rab33a and Rab33ba mediate the outgrowth of forebrain commissural axons in the zebrafish brain, Sci. Rep. 9:1799. (LINK)
- Nakazawa, H., Sada, T., Toriyama, M., Tago, K., Sugiura, T., Fukuda, M. and Inagaki, N. (2012)
Rab33a mediates anterograde vesicular transport for membrane exocytosis and axon outgrowth. J. Neurosci. 32, 12712?12725.(LINK)
- Toriyama, M., Sakumura, Y., Shimada, T., Ishii, S. and Inagaki, N.(2010)
A diffusion-based neurite length sensing mechanism involved in neuronal symmetry-breaking, Mol.Syst.Biol. 6, 394.(LINK)
- Mori, T., Wada, T., Suzuki, T., Kubota, Y. and Inagaki, N. (2007)
Singar1, a novel RUN domain-containing protein, suppresses formation of surplus axons for neuronal polarity, J. Biol. Chem. 282, 19884-19893.(LINK)
- Toriyama, M., Shimada, T., Kim, K-B., Mitsuba, M., Nomura, E., Katsuta, K., Sakumura, Y., Roepstorff, P. and Inagaki, N. (2006)
Shootin1: a protein involved in the organization of an asymmetric signal for neuronal polarization. J. Cell Biol. 175, 147-157.(LINK)